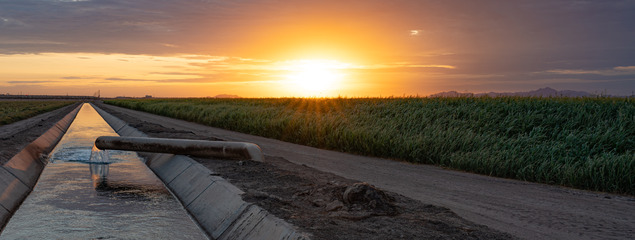
Increasing Irrigation Efficiency
Water Use and Management Research
Background & Literature Cited
Background
In arid regions, almost all agricultural crop water requirements come from irrigation. Irrigation water is applied to replace water lost from the crop rooting zone by transpiration through the crop leaf canopy, evaporation from the soil surface, and percolation below the rooting zone, before physiological stress occurs. The combined loss of water from the rooting zone by transpiration and evaporation is crop consumptive use or crop evapotranspiration (ETc).
Water Required for Crop Growth
The most comprehensive database of consumptive water use of crops in Arizona was generated by Erie et al., 1981. Erie and coauthors estimated crop water use by measuring soil water depletion in the crop rooting zone with temporal soil sampling and gravimetric water determinations. This approach assumes that after a certain soil moisture tension, drainage becomes minimal. This was the best method available to Erie et al. at the time, but the assumption is sometimes inaccurate. In reality, water continues to move in response to energy gradients, and there are uncertainties as to whether reductions in soil moisture in the crop rooting zones are due to evapotranspiration loss or continued drainage. Fortuitously, for the medium texture soils with deep water tables in central Arizona where Erie did this work, errors in ETc estimates to drainage were likely minimal. Perhaps, the greater limitation to the database of Erie et al (1981) is that the data are about 5 decades old and do not represent current cropping varieties and practices that have evolved over the past half century. Furthermore, the agricultural industry in Arizona currently includes several commodities that were not grown in Erie’s time and data for these crops do not exist as part of this database.
Another source for crop ETc estimates is FAO-56 (Allen et al., 1998). The authors of this document complied ETc data from around the world and provided a protocol to estimate crop ETc from growing period, crop coefficients (Kc) characteristic to each growing period, and reference evaporation (ETo). ETo is calculated from local weather data using the Penman-Monteith approach. The Kc values are specific by crop type and local climate and are determined experimentally from the ratio of measured ETc to ETo. While the database compiled by Allen et al. is comprehensive and robust, it was compiled from areas around the world and was intended as a starting point, and not as a substitute for locally calibrated data.
A third source for crop ETc is a modification of FAO-56: it is provided by the United States Bureau of Reclamation (USBR) , as part of their Lower Colorado River Annual Summary of Evapotranspiration and Evaporation (LCRAS) system (https://www.usbr.gov/lc/region/g4000/wtraccttypes.html). Whereas FAO-56 aligns crops coefficients with growth period by days, LCRAS estimates crop acreage and growth period using satellite imagery.
Considering all three methods, current ETc data for the complex multiple cropping systems in the irrigation districts near Yuma were lacking. While the methodologies were sound, all had shortcomings: they were based on data collected on farms remote from Yuma and thus may not be representative, and they relied upon generalized coefficients not specific to current Yuma crops. Furthermore, crop coefficients derived from the data of Erie and FAO 56 are tied to days
of cropping and in Yuma planting dates vary widely resulting in different period from planting to maturity. Therefore, our objective is to tie crops coefficients to other estimates of growth stage.
Growing Degree Days
Growing degree days (GDD) or heat units (HU) are used to predict growth and development of many crops. The concept assumes that growth only occurs if temperature exceeds some minimum threshold. The concept often also assumes that no further growth occurs above some maximum threshold. Each crop would have a characteristic minimum and maximum threshold.
A number of approaches have been proposed to calculate heat units. The simple approaches use a mean calculated from daily minimum and maximum temperatures and subtracting the minimum threshold. However, in cases where the calculated mean exceeds the maximum threshold, the minimum threshold is subtracted from the maximum threshold.
The AZMET weather system in Arizona generally uses the single sine curve approach (Brown. 2010; 2013). This approach involves forcing a sine curve through the daily minimum and maximum temperatures. The area bounded by the sine curve and lower and upper threshold temperatures represents the accumulated heat units.
For some crops, solar radiation, in addition to temperature, provides better predictions of crop growth stages (Wurr et al., 2002). The effective degree day (EDD) approach was developed the factor in the effects of solar radiation and has reported to provide more consistent predictions than GDD alone from some production scenarios (Scaife et al, 1987).
A major limitation of the GDD approach is the assumption that growth is largely driven by temperature and no other biotic or abiotic stresses are limiting growth. For the high value cropping systems in Yuma, growers generally manage crops to avoid these stresses, and this assumption would generally be applicable. However, when these stresses occur, the GDD would inaccurately forecast growth.
Remotely sensed indices of growth
There is a growing array of space-based platforms that allows for the quick estimation of vegetative indices (VI) that facilitate the tracking of growth. The most common index utilized is the normalized difference vegetative index (NDVI). It is calculated by calculating the difference between the near infrared band (NIR) and red band (Red) and dividing by the additive of NIR and Red bands. The USBR LCRAS system (https://www.usbr.gov/lc/region/g4000/wtraccttypes.html) and the SIMS routine of Open ET (https://openetdata.org//methodologies) currently use this approach. More recently, we have shown its utility in partitioning in-season crop coefficients (French et al., 2020).
As part of this research, we have explored alternative bands and indices, but with rare exceptions, the NDVI index provided satisfactory results. We also evaluated alternative platforms and found the Sentinel system provided adequate temporal and spatial resolution for the crop production systems we evaluated. The Sentinel system is also available without costs for access.
Perhaps the major limitation of the VI approach is the signal does not emerge from background until there is detectable canopy cover, but this would only be an issue in our application if a change in crop coefficient is required before that time. A second limitation is that cloud cover might interfere with the imagery. While this would infrequently be an issue in the Yuma area with 320 sunny days, it would be in many parts of the county.
Water Required for Salt Management
Beyond the water required for crop ET, there are other beneficial uses of water. These might include land preparation, residue decomposition, germination, irrigation to address distribution uniformity issues, frost control, and salt management. Salt management particularly is of paramount importance to sustainability in the arid agricultural areas of Arizona. Soil minerals, irrigation water, and shallow groundwater in much of the flood plain districts near Yuma contain salts that accumulate in the fine textured soils. Without management, salt concentrations would become too high to grow crops. Thus, some level of excess irrigation (beyond ETc) must be applied to leach salts below the crop root zone. Effective leaching is especially important in this region because many of the high value horticultural crops produced are sensitive to salinity (Sanchez and Silvertooth, 1996).
Leaching requirements are typically estimated using steady state mass balance assumptions (Rhoades et al., 1974). Steady state assumptions ignore salt uptake by plants and precipitation and dissolution reactions of carbonates in the root zone. Ignoring plant uptake results in insignificant errors because the amount of salt taken up by plants are small relative to the total salt concentration in the rooting zone. On the other hand, precipitation and dissolution reactions should not be ignored when water high in bicarbonate is used for irrigation. In these cases, transient modeling would be needed to avoid over-estimated leaching requirements (Corwin et al., 2007; Letey er al., 2011). However, the steady state approach remains the best approach for field practitioners since transient models are complex (Corwin and Grattan, 2018) and the input data for these models are not available across a wide range of soil types.
The leaching requirement in complex cropping systems is aimed toward the most salt-sensitive crop in the rotation. For the flood plain irrigation districts in Yuma this is lettuce, with a leaching requirement of 20% if irrigated with Colorado River water. For economic and environmental reasons, the required leaching for salt management is deferred to an off-season pre-irrigation. This has enabled better weed and disease management and reduced non-point source pollution from improved in-season management of N fertilizers, soil herbicides, and soil insecticides.
Literature Cited
- Allen, R., L. S. Pereira, D. Raes, and M. Smith. 1998. Crop evapotranspiration - Guidelines for computing crop water requirements - FAO Irrigation and drainage paper 56.
- Brown. P. W. 2010. Arizona heat unit program. Arizona Meteorological network. http://ag.arizona.edu/azmet.
- Brown P. W. 2013.Heat Units. Arizona Cooperative Extension Bulletin AZ1602.. Univ. of Arizona, Tucson, AZ
- Corwin, D. L., J. D. Rhoades, and J. Simunek. 2007. Leaching requirement for soil salinity control: Steady-state versus transient models. Ag. Water Mang. 90:165-180.
- Erie, L. J., O. F. French, D. A. Bucks, and K. Harris. 1981. Consumptive use of water by major crops in the Southwestern United States. Department of Agriculture Conservation Research Report no. 29.
- French, A. N., D. J. Hunsaker, C. A. Sanchez, M. Saber J. R. Gonzalez, and R. Anderson. 2020.
- Satellite-based NDVI crop coefficients and evapotranspiration with eddy covariance validation for multiple durum wheat fields in the US Southwest. Agriculture Water Management. 239:https://doi.org/10.1016/j.agwat.2020.106266
- Letey, J. G. J. Hoffman, J, W, Hopmans, S. R. Grattan, D. Suarez, D. L. Corwin, J. D. Olster, L. Wu, and C. Amrhein. 2011. Evaluation of soil salinity leching requirement guidelines. Agric, Water . Manage. 98:502-506. Open. ET. https://openetdata.org/methodologies/
- Rhoades J. D. and J. van Schilfgaarde (Ed.), Drainage for Agriculture. Am. Agron. Soc. Monogr., vol. 17 (1974), pp. 433-462
- Sanchez, C. A., and J. C. Silvertooth. 1996. Managing saline and sodic soils for the production of horticultural crops. HortTechnology 6:99-107.
- Scaife, P. J., E. F. Cox, and G. E. L. Morris. 1987. The relationship between shoot weight, plant density, and time during the propagation of four vegetable species. Ann. Bot. 59: 325-334.
- USBR. Colorado River Accounting and Water Use Reports. https://www.usbr.gov/lc/region/g4000/wtraccttypes.html
- Wurr, D.C. E., J. R. Fellows, and K. Phelps. 2002. Crop scheduling and prediction -Principles and opportunities with field vegetables. Adv. Agron. 76:201-234.